Plants as Bioreactors: The Next Frontier for Commercial-Scale Protein Production?
• Technology • Investment • Feature
The rise of recombinant technology
How is the human insulin protein to treat diabetes made? You can’t farm humans, so you use recombinant protein technology. This is the name given to proteins produced from the expression of recombinant DNA, which is DNA that has been created by combining DNA from at least two distinct sources. This DNA is expressed through a cell factory which is used to produce the recombinant proteins (or indeed theoretically any other complex molecule). In more simple terms, we can programme cells to become cell factories and spit out specific proteins as instructed. There are many different types of cells we can use as protein factories (also called expression systems): microorganisms (such as yeast, bacteria or algae), mammalian cells, insect cells, and plant cells, are all viable.
Recombinant technology has been used for decades. Humulin or recombinant insulin was the first commercially available recombinant protein produced in 1982. Since then, numerous proteins for pharmaceutical, food, feed, detergents, fuel, and other applications have been commercially produced. Today, more than 170 recombinant proteins for pharmaceutical applications are approved and produced globally.[i]
In the food industry, recombinant protein technology was initially focused on the production of enzymes and other food processing aids. Chymosin, used to coagulate milk in cheese production, is the most well-known example of using recombinant protein technology to improve food production efficiency, lower costs, and remove the need for animals to generate raw materials. More recently, the drive to create more sustainable, ethical, and efficient versions of meat, egg, and dairy products and other animal-derived products has resulted in the use of recombinant protein technology to produce functional ingredients. Impossible Foods’ soy leghemoglobin and Perfect Day’s whey are the first commercially available examples of recombinant proteins used in meat and dairy analogues respectively.
Not so simple cell factories
While thousands of proteins have been produced through recombinant technology in the lab for R&D purposes, the next step to scale and commercialize these processes has presented more of a challenge.
Two platforms have emerged with the most commercial potential for expressing recombinant proteins: 1) precision fermentation, where cells (usually microorganisms such as bacteria or yeast for the purposes of food) are grown in a bioreactor and the proteins are expressed and extracted; and 2) precision horticulture (sometimes called molecular farming or plant-produced proteins), where plant cells are used as the expression system and are grown as crops through indoor or outdoor farming, with the desired proteins ultimately extracted from the plants (See Exhibit 1). Through this article we will use the term precision horticulture for this production method.
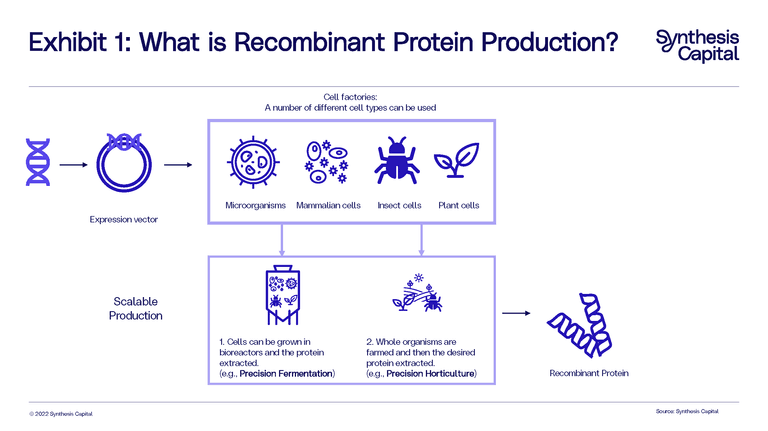
The primary difference between the two platforms lies upstream in how the different organisms are grown. With precision fermentation, microbes are grown in a large reusable stainless-steel bioreactor where nutrients (feedstock) and heat are provided to enable fermentation, cell growth, and protein production. Once the fermentation process is complete, the target protein is recovered from the cells or fermentation broth.
With precision horticulture, the plants are grown in fields, greenhouses, or vertical farms. In this process, the plant acts as a single-use bioreactor converting sunlight, CO2, fertilizer, and water into nutrients, which the cells in the plant use to produce the desired recombinant protein. To retrieve the target protein, the crop must be harvested and processed. The level and type of processing will depend on the type of and part of the plant used for protein production (i.e. the leaf of a lettuce plant versus the seed of a soybean plant). In cases where the crop is already commercially processed to produce a protein concentrate, such as soybean, the first stages of processing (e.g., milling, grinding), will be similar to the conventional process.
The final downstream purification steps to isolate a high purity recombinant protein from precision horticulture will likely be similar to those used in precision fermentation (e.g. centrifugation or chromatography). However, plant-derived recombinant proteins can also require additional purification steps to remove impurities and plant secondary metabolites.
Using plants as bioreactors is nothing new
Using plants as bioreactors has long been promised as a cost-effective and easily scalable strategy to produce high quality proteins, however, until recently, the pharmaceutical industry has had little success commercializing it. The first recombinant plant-derived pharmaceutical protein was human serum albumin in 1990, initially produced in transgenic tobacco and potato plants.[ii] Despite this early promise, precision fermentation ended up becoming the preferred route due to relatively low yields, inconsistent product quality, and the difficulties with downstream processing when using plants.[iii] In addition, regulatory inertia contributed to slow commercialization.
Today, there remain several primary hurdles to widespread commercialization of precision horticulture. Firstly, because the plants are genetically modified, in Europe there are legal restrictions on their growth. Secondly, downstream processing has continued to be an issue due to the cost associated with removing impurities from the plant system. Thirdly, with open field farming, there are concerns relating to cross contamination with other crops, especially for wind-pollinated plants.
But momentum is gathering, catalysed by recent rapid improvements in synthetic biology and a growing market for recombinant proteins in food applications – using an edible crop to produce a food ingredient has significant appeal over using an inedible microbe to do the same.
Traditionally, for precision horticulture, the time and cost required to develop, screen, and select a plant to produce a desired protein could be prohibitive. Now, applying modern gene editing technology can create better outcomes more rapidly and with greater yields. Indeed, using plants as bioreactors for pharmaceutical applications continue to show promising gains in protein production efficiency[iv] and is gaining traction and growing in cost competitiveness with precision fermentation, as evidenced by improvements in protein yields and production timelines.
This was supported by a 2016 study assessing the techno-economics of monoclonal antibody production via plants. By improving protein expression levels and optimizing downstream purification, the model predicted >50% reduction in cost of goods compared to published values for precision fermentation.[v]
In terms of commercial applications, the pharmaceuticals industry has been an initial focus. Vaccines are one such example: in February 2022, the biotech firm Medicago gained approval in Canada for its plant-grown COVID-19 vaccine Covifenz. Indeed, the theory that using plants to produce edible vaccines (for example in lettuce or other leafy crops) is an attractive one, offering an elegant solution of oral delivery and the elimination of downstream processing[vi] – especially when compared to other vaccine production methods such as in chicken eggs.[vii] There are now several companies using precision horticulture to produce recombinant growth factors for cell culture applications and other therapeutic applications (e.g. collagen).
However, just as we have seen for precision fermentation, this momentum is crossing over into food applications, with companies starting to plants to produce ingredients and raw materials for the food industry. We have more than twenty companies in our pipeline using precision horticulture to produce key proteins for use in food production. Some are focusing on proteins like casein and others on growth factors used in cell culture.
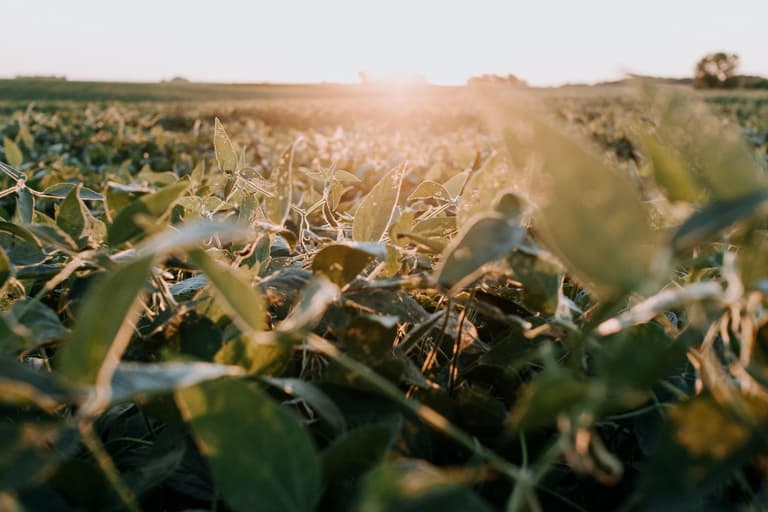
Precision horticulture or precision fermentation: pros and cons
Both platforms have advantages and disadvantages for commercial production of food ingredients. While precision horticulture is gathering momentum, technical and commercial innovations with precision fermentation for food applications are progressing rapidly. As a result, it is unlikely that there will be a single technology platform used to produce all recombinant ingredients in meat, egg, and dairy analogues. Rather the industry will select the most appropriate platform for a given protein based on scalability, cost, impact on end-product functionality, regulatory openness, and speed to market in each target market. (See Table 1).
Precision Fermentation offers a faster route to market in the short-term
Broadly, precision fermentation has significantly faster R&D timelines due to the rapid doubling times of microbes versus plants, meaning experiments can be accomplished in days rather than the months required for many commercial crops. Plant cell culture systems such as tobacco BY-2 cells, which have comparable doubling times to microbes, can be used but such systems lack the scalability of crops such as soy.
As a result, companies looking to commercialize multiple proteins that are less price sensitive will benefit from the flexibility the shorter R&D timelines microbial systems provide. In the long term, we may see companies test smaller markets via precision fermentation or rapid precision horticulture systems before moving to large-scale open field precision horticulture for blockbuster proteins required in massive quantities by food companies.
Precision horticulture offers a lower cost, less capex-intensive scale-up route
While many products are produced at scale with precision fermentation, the industry has not yet fully scaled up to produce very large quantities of food-grade ingredients. Building this large-scale manufacturing infrastructure is capex intensive. Meanwhile precision horticulture in open fields offers a potentially cheaper and faster route to producing much larger volumes of proteins. However, precision horticulture in greenhouse and indoor farming, which may be the only path to commercialization in some regions, either due to environmental or regulatory concerns, could face similar scale-up challenges as precision fermentation and may only be applicable to lower volume ingredients.
Lowering downstream processing costs and increasing yields will be key to lowering PH costs
Downstream processing is the main cost driver for plant derived proteins in the pharmaceutical industry as plant secondary metabolites and other impurities must be removed. For food applications, there are options to use consumable plants such as soy, pea, or lettuce to reduce purification steps, as well as providing a potential revenue generating side-stream, helping to offset manufacturing costs.
Precision horticulture also needs to increase recombinant protein yields in the plants themselves while maintaining the overall crop yield. We anticipate modern genetic engineering tools will help with this. Along with the scalability of open field precision horticulture, improving these means precision horticulture will benefit from the lower costs of large-scale open-field farming, resulting in production costs competitive with conventional animal proteins and lower than precision fermentation.
The primary cost drivers for commercial-scale precision fermentation are feedstock, energy, and downstream processing. Lower-cost carbon sources such as CO2, improved or novel strains that can produce proteins at significantly higher titres, and a shift to renewable energy have the potential to reduce costs. These innovations will take time and it is currently unclear whether they can improve production costs sufficiently to be competitive with open-field precision horticulture.
Precision horticulture offers a co-delivery option to improve end-product functionality
Both platforms can produce bioidentical proteins and other molecules. The research around which types of cells and organisms are best at producing which types of molecules is on-going (and further ahead microbial fermentation due to decades of research and investment by biopharma).
One area where precision horticulture may have an advantage is with the ability to deliver the recombinant protein along with other plant ingredients, such as proteins or fats. This co-delivery may enhance functionality and lower costs through reduced downstream processing.
The regulatory path for precision horticulture is more complex than precision fermentation
Most jurisdictions have established regulatory approval processes for ingredients and food produced from engineered microbes, with products approved in several countries.
In contrast, precision horticulture faces challenges on two fronts. First, multiple countries have restrictions on the farming and sale of genetically modified plants. Second, concerns surround potential contamination events of crops introducing allergens into the food supply chain. However, we are seeing movement with the US recently introducing a more streamlined approval process for genetically modified plants and other jurisdictions such as the UK and China becoming more open to GM crops.
In summary, both platforms are capable of producing proteins and other molecules that will positively impact end-product functionality in meat, egg, and dairy analogues, but, assuming its regulatory barriers can be overcome, currently precision horticulture has compelling cost and scale advantages compared to precision fermentation, especially for proteins required in large volumes. Due to its faster research and product development lifecycles, precision fermentation will continue to be useful for higher value ingredients required in lower volumes.
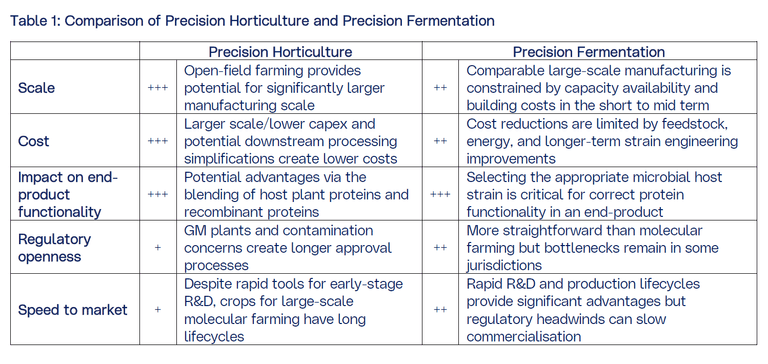
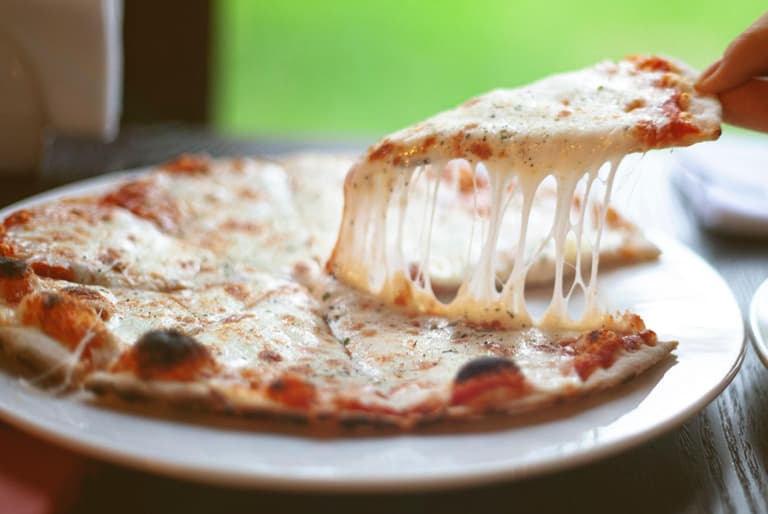
Applying precision horticulture to cheese without the cow
Cheese remains the holy grail for many alternative protein producers. Plant based cheeses have improved in recent years, but remain disappointing. Companies have struggled to achieve the functionality (i.e. stretch and melt) and taste of dairy cheese using plant-based ingredients.
These qualities are down to a vital dairy protein found in milk: casein. Indeed approximately 80% of the protein found in milk is casein, and the remaining 20% is whey. While we refer to casein protein as one protein, it is actually a family of molecules (phosphoproteins) and it is these casein proteins that are vital in the production of cheese.
Casein production presents a unique challenge. Casein proteins exist as aggregates in milk called casein micelles (See Exhibit 2). The behaviour of these micelles under different conditions forms the essential component of conventional cheese production, and provides the functional properties that create the stretch, meltability, texture, mouthfeel, and other sensory attributes that consumers associate with great tasting cheese – and which plant-based cheeses have been, so far, unable to recreate. It is challenging to recreate micelles and micelle-fat interactions with plant proteins. This is unlike whey proteins, where production of the main protein β‑lactoglobulin (BLG) provides the functionality needed; in essence the production process can stop at the tertiary protein structure shown in Exhibit 2.
In a full circle moment, the final stage of cheese making is the addition of the enzyme chymosin (one of the first enzymes to transition to production via fermentation!) to milk, which facilitates the production of cheese by causing the micelles to aggregate into the gel-like cheese curd.
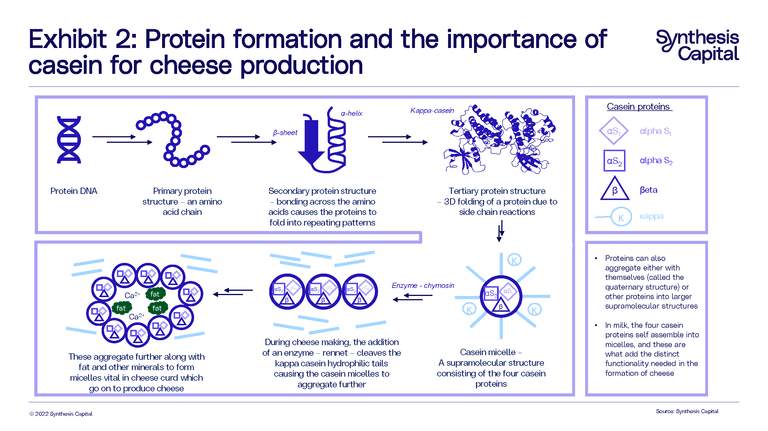
Whey protein produced via precision fermentation from Perfect Day is available through consumer products in the US today (via consumer products like MyProtein’s protein powder, the CO2COA™ chocolate bar with Mars, and Brave Robot ice-cream). However, food-grade precision fermented casein proteins are not yet available commercially at the scale required for food. This is because forming the casein micelles has added a layer of complication to commercialization. Two to four specific casein proteins are needed to form micelles so these need to be produced and, for most companies focusing on precision fermented casein, assembled into micelles outside the cell factory – resulting in a higher cost. Today, there are more than 20 companies focusing on precision fermentation of casein molecules.
The production of casein is one example of where we believe precision horticulture could play an important role. This is for two primary reasons. Firstly, the complexity of casein proteins and the micelle structure means it is a challenging molecule to produce on a commercially relevant scale through precision fermentation. Secondly, the large volumes of casein required for cheese production given it is the majority protein in milk, means that the potential scalability of precision horticulture is very interesting. Currently, we believe the cost and scale constraints of producing casein proteins and casein micelles via precision fermentation – particularly when using traditional sugar-based feedstocks and existing fermentation technology – will make it challenging, if not impossible, for precision fermentation to compete on price with field-based precision horticulture.
Mozza Foods' unique approach to produce real dairy cheese from plants
Mozza Foods, based in California, is an example of a company in this sector with a unique and patented approach to apply precision horticulture to the micelle challenge: forming the casein micelles inside the plant cell factories. While more challenging from a molecular biology standpoint, this strategy has the potential to streamline production (one plant instead of several) and downstream purification (casein micelles are an order of magnitude larger than individual casein proteins and thus easier to purify), thus enabling lower cost casein micelle production.
Mozza’s primary focus will be to use the casein produced in mozzarella cheese – in part because of mozzarella’s simpler structure and also because it is a nearly $50 billion global industry.[viii] Mozza will initially target the US mozzarella market, which is currently valued at around $10 billion, and has a better-defined regulatory process and established market for recombinant protein ingredients. In the US, we estimate almost 10% of cheese consumed is as a pizza-topping.[ix] Ultimately however, there is no reason why Mozza’s caseins cannot be applied to any produce any cheese.
In the US almost 10% of cheese consumed is on pizza
Precision horticulture as the next frontier for commercial protein production
Mozza are not the only precision horticulture company looking at casein proteins. Other companies, such as Nobell Foods, Imagene, and Miruku are just some of the precision horticulture companies working on casein. Overall, we expect the investment and interest in precision horticulture as a production method to increase, given its potential advantages for commercial scale production, particularly for more complex proteins such as caseins. We believe that Mozza’s differentiated approach of growing the casein micelles in vivo is a particularly promising one, and we are excited to continue our partnership with them after having led their Series A round earlier this year.
[i] Pham P V. (2018) Chapter 19 - Medical biotechnology: Techniques and applications. Omics Technologies and Bio-engineering 1:449-469. Please find here.
[ii] Sijmons PC, Dekker BM, Schrammeijer B, Verwoerd TC, van den Elzen PJ, Hoekema A (1990) Production of correctly processed human serum albumin in transgenic plants. Biotechnology (NY) 8: 217–22. Please find here.
[iii] Front. Plant Sci., 11 June 2019 Sec. Plant Biotechnology Link here.
[iv] Fischer R, Buyel JF. Molecular farming – The slope of enlightenment. Biotechnology Advances. 2020;40(August 2019):107519. doi:10.1016/j.biotechadv.2020.107519
[v] Nandi, Somen, Aaron T. Kwong, Barry R. Holtz, Robert L. Erwin, Sylvain Marcel, and Karen A. McDonald. “Techno-Economic Analysis of a Transient Plant-Based Platform for Monoclonal Antibody Production.” MAbs 8, no. 8 (November 16, 2016): 1456–66. Link here..
[vi] Shanmugaraj B, I Bulaon CJ, Phoolcharoen W. Plant Molecular Farming: A Viable Platform for Recombinant Biopharmaceutical Production. Plants (Basel). 2020 Jul 4;9(7):842. doi: 10.3390/plants9070842. PMID: 32635427; PMCID: PMC7411908.
[vii] Rajaram, S., Boikos, C., Gelone, D. K. & Gandhi, A. Influenza vaccines: the potential benefits of cell-culture isolation and manufacturing. Ther. Adv. Vaccines Immunother. Link here. (2020).
[viii] Inc, G. I. A. (n.d.). Global Mozzarella Cheese Market to Reach $47.3 Billion by 2026. Link here.
[ix] Synthesis Capital analysis